1.IntroductionIt is known that hyperglycaemia changes red blood cell membrane fluidity1 2 3 4 5 and impairs red cell deformability.6 7 Evaluation of the amount of glycated hemoglobin in erythrocytes could be an early diagnostic marker of hyperglycemia. An easily accessible red blood cell (RBC) characteristic is its refractive index,8 9 10 which is due to the amount of hemoglobin it contains, since it is about 95 of the erythrocyte weight. Using polarizing-interference microscopy it is possible to measure the light refractive index in separate erythrocytes. Additional information about the hemoglobin amino acid content can be obtained by measuring the refractive index of erythrocytes at different pH levels.11 Each amino acid molecule contains an amino group (NH 2) at one end and a carboxyl group (COOH) at the other end, and different side groups (R). R groups are classified according to their polarity: (1) apolar or hydrophobe R groups, (2) uncharged polar R groups, (3) “+” charged R groups, (4) “−” charged R groups. In neutral solutions amino acids are doubly charged, but overall are neutral. In these circumstances molecules have high dielectric permeability and large dipole moments. These amino acid qualities are similar in protein solutions. Moreover, each protein has only one amino group and one carboxyl group, but several different R groups. Classical theory of light dispersion in fluids can be applied to a cell. According to this theory, the light refractive index depends on the light’s wavelength or on the frequency of light waves. There is a relationship between the polarizability of an atom or a molecule and the light refractive index. In general, if material consists of molecules of different polarizability, the total polarizability can be obtained as the sum of all individual molecules’ polarizability. It is necessary to take into account that the molecule is affected by the external electric field and by electric fields induced by neighboring molecules.12 According to light dispersion theory, the light refractive index formula is as follows: where ε is material permeability, N the number of molecules, m the molecule’s mass, q the molecule’s charge, ω the oscillation frequency, ωo the oscillation self-frequency, and 2γ=g/m the force index, similar to friction force. This formula suggests that protein R group charge can affect the value of the refractive index regardless of its charge sign. Dissociation of R group protein molecules describes each protein and the refractive index (RI) depends on the content of charged amino acids in the molecule.The aim of this study was the qualitative and quantitative evaluation of the hemoglobin of separate erythrocytes in diabetic patients and in healthy donors. To do this we have elaborated on a new optical method for hemoglobin characterization in intact and fixed cell erythrocytes.13 14 2.Material and Methods2.1.Polarizing-interference Microscopy of RBCTwo kinds of RBCs, fixed and intact, were investigated. Smears of PB were prepared on slides, air dried, fixed for 3 min in methanol and air dried again. Mixtures of polyvinylpyrolidine and buffers of different pH (1:1) were used as embedding media. The RI of the embedding media was stable (n=1.5133±0.0001) and independent of the type and the pH of the buffer. It was used as a standard value for the evaluation of the RI at different pH levels. Smears were embedded in the buffered medium and the refractive index for 35 RBCs was measured at each of the subsequent 18 different pH levels in the range of pH=2–13. Intact PB was mixed with buffered embedding media and overlaid with a coverslip just before being investigated. Intact RBC refractive index measurements were carried out in a manner analagous to those with fixed cells. A Nomarski polarizing-interference microscope MPI-5 (Poland) was used for the measurements of light phase retardation. Using a Wollaston prism mounted on an objective the erythrocyte images for ordinary and extraordinary light beams were completely separated. In the thickest erythrocyte region the first interference maxima were visually adjusted to the eye sensitive purple color for ordinary and extraordinary images by shifting the second Wollaston prism placed on the objective’s rear focus. For each erythrocyte measured the Wollaston prism displacement rendered a second value. From the whole interference band width h and measured Wollastone prism displacement 2d the phase retardation ϕ was calculated for each erythrocyte. Measurements were carried out at white light with an arbitrary wavelength of λ=0.550 μm. The value of the erythrocyte refractive index was calculated from the light phase retardation using where nv=1.5133±0.0001 refractive index of the embedding media. Deviation d and interference band width h=2.71 were obtained from the Wollaston prism shifting in millimeters. In the first step the RBC thickness was estimated using two embedding media with different n ( nv1 and nv2 ) and the measuring phase deviation for fully divided RBC images. Full RBC image duplication was acquired using both Wollaston prisms of the microscope objective and the prism between the objective and the ocular. The thickness of the erythrocyte was calculated as Using the RBC thickness measured, the refractive index was calculated for each pH value with a standard error of n±0.0005 and pH±0.05.3.Results3.1.Refractive Index of RBCs3.1.1.Refractive Index of Fixed CellsCurves of the refractive index were obtained for nine diabetic patients and seven healthy donors. For each individual, the refractive index values determined at 18 different pH levels are depicted, in Figure 1(a) for the diabetics patients and in Figure 1(b) for the control group. The RI curves of both groups were of similar configuration, with one branch in the acid portion of the pH scale (the acidic branch), a maximum (the isoelectric point) and two minima in the neutral portion of the pH scale, and one branch in the alkaline portion of the scale (the alkaline branch). The curves of the individuals from the control group overlap each other, especially the isoelectric point and the alkaline branches [Figure 1(b)]. To the contrary, the curves of the diabetic patients revealed a distinct dispersion of their middle parts in comparison to one another within this group [Figure 1(a)]. Moreover, the middle portion (in the neutral pH zone) of the curves of the diabetic patients was shifted towards the alkaline end of the pH scale in comparison with the control curves. The isoelectric point in diabetic patients corresponded to pH=7.1, whereas the isoelectric point in the control group was pH=6.5. In healthy donors the refractive index was in the range of n=1.58–1.68, but the refractive index values in diabetic patients were lower (n=1.54–1.66). 3.1.2.Refractive Index of Intact CellsIntact RBC refractive index curves were obtained for four diabetic patients and four healthy donors. We observed differently shaped refractive index curves for intact RBCs of healthy donors [Figure 2(b)], with one deep minimum in the middle part (at pH=7.4 ) of the RI curve. However, the shape of the refractive index curve for intact RBCs in diabetic patients [Figure 2(a)] is similar to the RI curve for fixed RBCs in diabetics. 3.2.RBC Refractive Index Relationship with HyperglycaemiaPearson correlation coefficients were calculated at each pH between the RI values and the blood glucose concentration (Table 1) for nine diabetic patients (Table 2). A negative correlation tendency between the RI and the blood glucose concentration at the acidic and neutral zones of the RI curve was obtained, but a positive correlation tendency was observed between the RI and the blood glucose concentration in the alkaline zone of the RI curve. Table 1
Table 2
4.DiscussionThe data clearly demonstrate that the refractive properties of the RBCs in diabetic patients differ greatly from those of healthy donors. Among members of the control group, the refractive index maxima are located close together, with small deviations, which strongly suggests a uniform fixed RBC protein content [Figure 1(b)]. Significant deviation and depression of the RI curves for fixed RBCs were observed in the diabetic patients [Figure 1(a)]. The relationship between the refractive index and pH level can be explained by classic dispersion theory.12 We observed three maximum values of the refractive indices of RBCs along the pH scale, where the protein charge is larger due to the dissociation of basic (arginine or lysine) or acid (aspargine or glutamic acid) R groups. This suggests that the influence of amino groups and carboxyl groups on the refractive index is relatively small. The concentration of charged R groups that interfere with light (without reference to the sign) is an important factor in determining the refractive index. The significantly decreased RI value for the diabetic group can be explained by the strong interaction of glucose with hemoglobin. In addition, from the light refractive index formula, Eq. (1), we can see that RI value is inversely proportional to the molecule’s mass, and since the molecular mass of glycated hemoglobin is now increased, the RI value is smaller [Figure 1(c)]. The negative correlation between the blood glucose concentration and RI in the acidic portion of the RI curves supports this point of view (Table 2). The absence of a correlation in the alkaline end of the pH scale could be due to partial dissociation of glycated hemoglobin, leading to greater exposure of the hemoglobin molecule’s aspargine or glutamic acid charged R groups to the light electromagnetic field. Although the increasing charge of R groups increases RI [see Eq. (1)], at the same time the increased mass of glycated hemoglobin molecules decreases the RI. Since the influence of R-group charge is stronger than the mass of glycated hemoglobin molecules, the overall effect is to increase the refractive index. The intact RBC curve minimum in healthy donors [Figure 2(b)] could be associated with RBC membrane resistance to the buffered embedding medium in an attempt to maintain an intracellular pH and salt balance. Under such conditions RBC hemoglobin preserves the native zero-sum charged state. As a result the diabetic patients’ glycated hemoglobin RI value in the neutral pH region is the same as that in the fixed RBC case. In an acidic or alkaline environment the RBCs may lose membrane integrity, and hemoglobin may show similar erythrocytes RI properties upon glucose dissociation. REFERENCES
S. Eckarbom
,
Y. Bergqvist
, and
J. O. Jeppsson
,
“Improved method for analysis of glucated heamoglobin by ion exchange chromatography,”
Ann. Clin. Biochem. , 31 355
–360
(1994). Google Scholar
L. Mazzanti
,
E. Faloia
,
R. A. Rabini
,
R. Staffolani
,
A. Kantar
,
R. Fiorini
,
B. Swoboda
,
R. De Pirro
, and
E. Bertoli
,
“Diabetes mellitus induces red blood cell plasma membrane alterations possibly affecting the aging process,”
Clin. Biochem. , 25
(1), 41
–47
(1992). Google Scholar
S. I. Muzulu
,
R. F. Bing
,
R. I. Norman
, and
A. C. Burden
,
“Human red cell membrane fluidity and calcium pump activity in normolipidaemic type II diabetic subjects,”
Diabetic Med. , 11
(8), 763
–770
(1994). Google Scholar
R. A. Rabini
,
R. Galassi
,
R. Staffolani
,
M. Vasta
,
P. Fumelli
, and
L. Mazzanti
,
“Alteration in
Na+/K(+)
-ATPase activity and fluidity of erythrocyte membranes from relatives of insulin dependent diabetic patients,”
Diabetes Res. , 22
(1), 33
–40
(1993). Google Scholar
J. Fujita
et al.;,
“Nisoldipine improves the impaired erythrocyte deformability correlated with elevated intracellular free calcium-ion concentration and poor glycaemic control in NIDDM,”
Br. J. Clin. Pharmacol. , 47
(5), 499
–506
(1999). Google Scholar
T. Kunt
,
S. Schneider
,
A. Pfuetzer
,
K. Goitum
,
M. Engelbach
,
B. Schauf
,
J. Beyer
, and
T. Forst
,
“The effect of human proinsulin C-peptide on erythrocyte deformability in patients with type I diabetes mellitus,”
Diabetologia , 42 465
–471
(1999). Google Scholar
J. Beuthan
,
O. Minet
,
J. Helfmann
,
M. Herrig
, and
G. Mu¨ller
,
“The spatial variation of the refractive index in biological cells,”
Phys. Med. Biol. , 41
(3), 369
–382
(1996). Google Scholar
D. W. Leonard
and
K. M. Meek
,
“Refractive indices of the collagen fibrils and extrafibrillar material of the corneal stroma,”
Biophys. J. , 72 1382
–1387
(1997). Google Scholar
J. Wen
,
T. Arakawa
, and
J. S. Philo
,
“Size-exclusion chromatography with on-line light-scattering, absorbance, and refractive index detector for studding proteins and their interactions,”
Anal. Biochem. , 240
(2), 155
–166
(1996). Google Scholar
G. Mazarevica
and
T. Freivalds
,
“Proc. of the 11th Nordic–Baltic Conference on Biomedical Engineering,”
255
–256
(1999). Google Scholar
G. Mazarevica
,
T. Freivalds
,
R. Bruvere
,
N. Gabruseva
,
A. Leice
, and
T. Zvagule
,
“Proceedings of BIOS 2000,”
163
–171
(20002000). Google Scholar
|
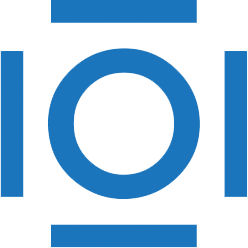
CITATIONS
Cited by 65 scholarly publications and 4 patents.