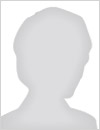
This will count as one of your downloads.
You will have access to both the presentation and article (if available).
The realization of the Ariel’s telescope is a challenging task that is still ongoing. It is an off-axis Cassegrain telescope (M1 parabola, M2 hyperbola) followed by a re-collimating off-axis parabola (M3) and a plane fold mirror (M4). It is made of Al 6061 and designed to operate at visible and infrared wavelengths. The mirrors of the telescope will be coated with protected silver, qualified to operate at cryogenic temperatures.
The qualification of the coating was performed according to the ECSS Q-ST-70-17C standard, on a set of samples that have been stored in ISO 6 cleanroom conditions and are subjected to periodic inspection and reflectance measurements to detect any potential performance degradation. The samples consist of a set of Aluminum alloy Al 6061-T651 disks coated with protected silver.
This paper presents the results of the morphological characterization of the samples based on Atomic Force Microscopy (AFM) and the reflectivity measurement in the infrared by Fourier Transform Infrared (FTIR) spectroscopy.
ARIEL is based on a 1 m class telescope feeding two instruments: a moderate resolution spectrometer covering the wavelengths from 1.95 to 7.8 microns; and a three-channel photometer (which also acts as a fine guidance sensor) with bands between 0.5 and 1.2 microns combined with a low resolution spectrometer covering 1.25 to 1.9 microns. During its 3.5 years of operation from an L2 orbit, ARIEL will continuously observe exoplanets transiting their host star.
This paper presents an overall view of the integrated design of the payload proposed for this mission. The design tightly integrates the various payload elements in order to allow the exacting photometric stability targets to be met, while providing simultaneous spectral and photometric data from the visible to the mid-infrared. We identify and discuss the key requirements and technical challenges for the payload and describe the trade-offs that were assessed during phase A, culminating in the baseline design for phase B1. We show how the design will be taken forward to produce a fully integrated and calibrated payload for ARIEL that can be built within the mission and programmatic constraints and will meet the challenging scientific performance required for transit spectroscopy.
The high sensitivity requirements of the mission need an extremely stable thermo-mechanical platform. In this paper we describe the thermal architecture of the payload and discuss the main requirements that drive the design. The ARIEL thermal configuration is based on a passive and active cooling approach. Passive cooling is achieved by a V-Groove based design that exploits the L2 orbit favorable thermal conditions. The telescope and the optical bench are passively cooled to a temperature close to 50K to achieve the required sensitivity and stability. The photometric detectors are maintained at the operating temperature of 50K by a dedicated radiator coupled to cold space. The IR spectroscopic channel detectors require a lower temperature reference. This colder stage is provided by an active cooling system based on a Neon Joule-Thomson cold end, fed by a mechanical compressor, able to reach temperatures lower than 30K.
Thermal stability of the telescope and detector units is one of the main drivers of the design. The periodical perturbations due to orbital changes, to the active cooling or to other internal instabilities make the temperature control one of the most critical issues of the whole architecture. The thermal control system design, based on a combination of passive and active solutions aimed at maintaining the required stability at the telescope and detector stages level, is described.
We report here about the baseline thermal architecture at the end of the Phase A, together with the main trade-offs needed to enable the ARIEL exciting science in a technically feasible payload design. Thermal modeling results and preliminary performance predictions in terms of steady state and transient behavior are also discussed.
During its four-years mission, ARIEL will observe several hundreds of exoplanets ranging from Jupiter- and Neptune-size down to super-Earth and Earth-size with its 1 meter-class telescope.3 The analysis of spectra and photometric data will allow to extract the chemical fingerprints of gases and condensates in the planets atmospheres, including the elemental composition for the most favorable targets. It will also enable the study of thermal and scattering properties of the atmosphere as the planet orbits around its parent star.
Taking advantage of emerging technology in mirror manufacturing and spacecraft formation flying, Simbol-X will push grazing incidence imaging up to ~ 80 keV and beyond, providing a strong improvement both in sensitivity and angular resolution compared to all instruments that have operated so far above 10 keV. This technological breakthrough will open a new highenergy window in astrophysics and cosmology. Here we will address the problematic of the development for such a distributed and deformable instrument. We will focus on the main performances of the telescope, like angular resolution, sensitivity and source localization. We will also describe the specificity of the calibration aspects of the payload distributed over two satellites and therefore in a not “frozen” configuration.
View contact details
No SPIE Account? Create one